Advanced Materials & Processing
The Advanced Materials & Processing Branch expand the engineering design space by creating new materials through synthesis, processing and fabrication that lead to enabling technologies that support current and future NASA missions. The area of materials synthesis involves creating new materials through composition of matter. This area has extensive chemistry and physics labs that allow for chemical, biochemical and polymer synthesis and purification, ceramics processing, and creating nanostructured materials.
Advanced Measurements & Data Systems
NASA Langley’s Advanced Measurements and Data Systems Branch (AMDSB) is responsible for research and development of experimental measurement and sensing techniques for aerospace research applications.
Aeroacoustics
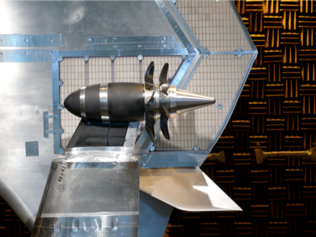
Counter-rotating propfan install above BWB airframe
The Aeroacoustics Branch plans and conducts research aimed at understanding and accurately predicting the noise from any air vehicle configuration, as well as defining and demonstrating concepts for air vehicle noise reduction. The scope of this research is broad and includes fundamental, theoretical, analytical, experimental, and applied research in aeroacoustics. The Aeroacoustics Branch directly supports multiple programs and projects projects within the NASA Aeronautics Research Mission Directorate.
Research emphasis includes the fluid mechanics and acoustics of various aircraft components such as high lift devices, landing gear, rotors, propellers/open rotors, and nacelles/liners. The interactions of various components, particularly propulsion airframe aeroacoustic effects (jet with airframe or propeller/rotor with airframe) including acoustic scattering/shielding, and atmospheric sound propagation are additional important areas of study. These activities lead to system level aircraft noise understanding, assessment, prediction, and auralization research efforts within the branch.
Objectives of the research are to understand aeroacoustic noise generation processes, to develop methods for predicting acoustics and flow fields and their interactions, and to identify and demonstrate noise reduction and control techniques. Experimental research is conducted in laboratories, in anechoic facilities, in wind tunnels, and on vehicles in flight. Current code development is based on Computational Fluid Dynamics in conjunction with the Lighthill acoustic analogy. The new multi-fidelity Aircraft Noise Prediction Program (ANOPP2) provides a framework that includes the workhorse ANOPP and its latest updates, as well as capability to utilize high-fidelity, physics-based tools. The NASA Auralization Framework (NAF) auralizes sounds from known source data allowing for perception influenced design.
Aeroelasticity
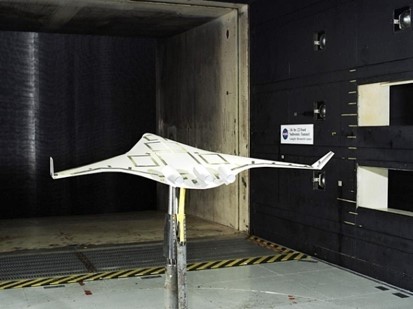
Blended Wing Body undergoing flight dynamics, stability, and control testing
The Aeroelasticity Branch potentially carries the responsibility of all things aeroelastic for lifting surfaces and airframes. Aeroelasticity by its nature encompasses interactions of structures (stiffness), mass properties (inertia), and aerodynamics, with occasional further considerations for thermal and propulsion effects. The Branch has a rich history of aeroelastic experimentation, analyses, and computational accomplishments. Aeroelastic loads and performance data have been acquired for many helicopter rotor-blade systems. Other types of vehicles assessed by the Branch include supersonic transports, hypersonic aircraft, and reentry parachutes and inflatable ballutes. The branch has influenced and enabled aeroelasticity understanding for decades running.
Aerothermodynamics
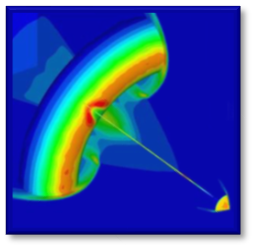
The towed toroidal ballute, a radical new hypersonic decelerator
The Aerothermodynamics Branch assess the flyability and survivability of aerospace vehicles as they fly through or re-enter the Earth’s atmosphere as well as the atmospheres of other planets. In order to perform this mission, the branch has a mix of experimentalists and computationalists who develop and apply aerothermodynamic tools that predict aerodynamic loads, surface aeroheating, and flowfield fluid dynamics — typically for high speed environments. Primary tools consist of force/moment and phosphor thermography global heating measurements along with LAURA and FUN3D Navier-Stokes CFD and D.
Applied Acoustics
The Applied Acoustics Branch focuses on the interaction of sound with structures and the interaction of sound with people. This includes aircraft interior noise, psychoacoustics, and acoustic liners for turbofan engines. Aircraft interior noise prediction and control requires an understanding of the manner in which sources of noise (e.g. turbulent boundary layer, jets, and rotors) interact with the fuselage that in turn interacts with the interior volume. Active and passive noise control methods are studied, utilizing an array of test facilities and test articles, including an occasional flight test. The interaction of sound with the interior surfaces of aircraft engines provides an opportunity for noise reduction in a challenging environment, including very high sound pressures, high flow speeds, and constraints on structural design and absorbing materials. In psychoacoustics, the Branch utilizes unique test facilities to improve our understanding of human perception of sound. These facilities include two sonic boom simulators which are used to study the acceptability of low level sonic booms, also called sonic thumps, that will be generated by next generation supersonic vehicles. The Branch also has an exterior sound simulator, which is used to study audibility and acoustic perception of aerial vehicles, current and future, that may be encountered in our nation’s communities.
Autonomous Integrated Systems Research
The Mission of the Autonomous Systems Integrated Research Branch is to conduct fundamental and applied research on integrated complex cyber-physical-human systems to develop, evaluate, and demonstrate efficient, resilient, and trustworthy autonomous systems and trustworthy collaboration between humans and autonomous machines; supported by rigorous physics-based modeling, explainable computational decision making, appropriate cognitive science techniques, and computational and physical experimentation.
Configuration Aerodynamics
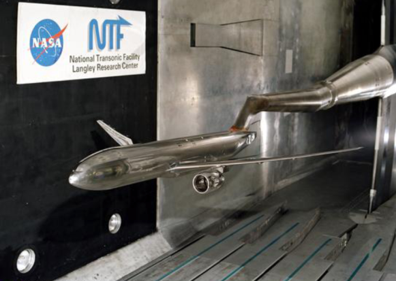
Pathfinder model used for tunnel calibrations and checks
The Configuration Aerodynamics Branch conducts applied experimental and computational research focused on the development of advanced configuration concepts for all vehicle classes at subsonic, transonic, and supersonic speeds. The emphasis of this research is to conceive and evaluate innovative aircraft platforms, control effectors, and propulsion system installations and assess the suitability for further development. This research is coupled with the development of an understanding of the flow physics and integrated aerodynamics. The experimental research is conducted using state-of-the-art analysis and experimental techniques in appropriate ground-based facilities and is focused at developing an understanding of flow physics associated with, and processes for, extrapolating to flight conditions the experimental results obtained in conventional wind tunnels.
Computational Aeroscience
The Computational AeroSciences Branch performs computational research in aerodynamics and acoustics with applications in all speed regimes, from subsonic to hypersonic flight. The Branch works to improve fundamental understanding of physics associated with the fluid mechanics and noise generation for complex airframe systems. The Branch conducts basic and applied research for improving the physical understanding of advanced techniques and models for the prediction and control of turbulent flows, with an emphasis on the high Reynolds number flows encountered on full-scale aircraft configurations.
Crew Systems & Aviation Operations
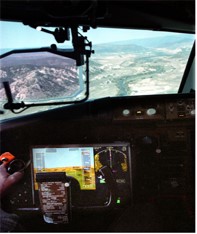
Flight test for Advanced Sensing, Avionics, Crew Research, and NAS Improvement
The Crew Systems and Aviation Operations Branch conducts fundamental and applied research, development, test and evaluation to support Aeronautics, Exploration, and aviation industry in developing the Next Generation Air Transportation System and beyond. Research efforts include: (1) development of concepts, technologies and procedures to increase en route and terminal airspace and surface capacities, (2) development of concepts and technologies to enhance flight safety and efficiency through the use of advanced flight deck technology including synthetic and enhanced vision, (3) modeling of aircraft wake turbulence enabling advanced wake-informed spacing concepts, (4) automated separation techniques that may allow manned and unmanned aircraft unfettered access to the National Airspace System, (5) assessment techniques for human crew awareness, engagement, fatigue, workload, etc. in multi-agent systems and (6) methods for measuring, predicting and optimizing function allocation and automation adaptation from a holistic system perspective.
Durability, Damage Tolerance, & Reliability
The Durability, Damage Tolerance, and Reliability Branch (DDTRB), as part of the Structures and Materials technology area, conducts a broad-based research and technology program that quantifies behavior, durability, and damage tolerance of structural materials; develops efficient, physics-based analytical and computational methods; develops new innovative test methods; develops radiation analysis and design tools for assessing the radiation environment; and validates performance of advanced materials and structures for aerospace applications in support of NASA, other government agencies, and the aerospace industry.
Dynamic Systems & Control Branch
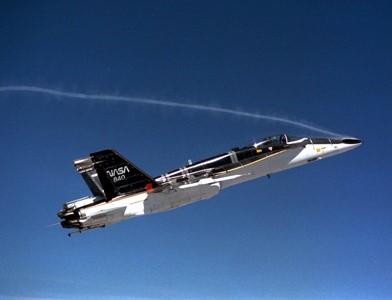
High-Alpha Research Vehicle (HARV) demonstrating high-alpha flight and advanced control concepts
The Dynamic Systems and Control Branch conducts applied and fundamental research in Controls, Guidance, Modeling & Simulation and System Identification. Research efforts include: (1) adaptive control of nonlinear systems, specifically systems under failure and damage conditions and control of unstable flight vehicles with significant levels of flexibility, rapidly changing mass properties and substantial uncertainties; (2) modeling of uncertain systems using stochastic techniques to properly account for real-world uncertainties that are inherent in all model-based control system design and analysis; (3) modeling of nonlinear vehicle dynamics due to separated flow and time dependent effects arising from rapid maneuvering and flow angles beyond the normal flight envelope, as well as the associated experiment design for nonlinear/unsteady modeling; (4) real-time system identification methods and application to distributed systems; (5) nonlinear flight simulation modeling exchange standards; (6) investigation of the pilot/vehicle interaction issues associated with varying levels of vehicle autonomy and requirements for bi-directional communications between pilot and vehicle; (7) development of advanced trajectory optimization techniques; (8) detection, mitigation and prevention of aircraft loss-of-control and (9) autonomy in unpiloted air and spacecraft.
Electromagnetics & Sensors
The Electromagnetics and Sensors Branch establishes Intelligent Sensory Systems throughout the entire electromagnetic/optical spectrum by pioneering in flight vehicle assessments of (remote) sensing technologies and methodologies that enable full perception of planetary and Earth atmospheric flight environments.
Flight Dynamics
The Flight Dynamics Branch works in the fields of attached and separated-flow (non-linear) aerodynamics, static and dynamic stability, control effector characteristics, dynamic modeling methods, application of flight control laws, flying and handling qualities, agility and maneuverability, and out-of-control flight characteristics. The Branch’s mission is to advance technology for the understanding and prediction of vehicle flight-dynamic characteristics; identify and provide solutions to difficult flight dynamics problems; and support development of new flight vehicle technologies. This research is performed through formulating, conducting, analyzing, and correlating of a wide range of research methods including static and dynamic wind tunnel tests, computational aerodynamics studies, dynamically-scaled model tests, analytical analyses, piloted simulation studies, and flight tests. The Branch has traditionally been focused on atmospheric flight vehicles – including those that are transiting through the atmosphere on launches and/or entry vehicles. A current research focus is development of self-learning technologies for reducing new-vehicle development cycle time and for enabling increased autonomy in flight vehicles.
Flow Physics & Control
The Flow Physics and Control Branch investigates the fundamental physics of unsteady viscous flows across the full speed regime, from subsonic through hypersonic speeds, using experimental and computational tools. The Branch conceives, formulates, and conducts experimental investigations with the goal of transitioning physical understanding to engineered systems. Cutting-edge techniques and methods are used to explore, analyze, and exploit complex 3-D phenomena in quasi-steady and unsteady environments with multiple scale physics, such as turbulent, transitional, and separated boundary layers. This information is also used to develop and advance physics-based computational tools and methods in close coordination with the computational fluid dynamics community. In addition, the Branch develops and invents flow control technologies and strategies to liberate aircraft designers from flight envelope constraints. Innovations in flow control are discovered in the laboratory through the study of unsteady aerodynamics coupled with control theory and actuator behavior and demonstrated at flight scales. Recent FPCB research includes developing approaches for simplified high-lift systems, controlling separated flows, leveraging vortical flows, controlling boundary layer transition, and reducing turbulent viscous drag.
Hypersonic Airbreathing Propulsion
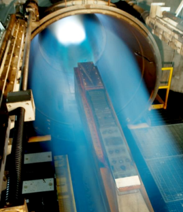
X-51 in 8FT HTT
The Hypersonic Airbreathing Propulsion Branch (HAPB) performs multidisciplinary research to develop advanced technology for hypersonic airbreathing propulsion systems for aerospace vehicles, focusing on airframe-integrated engine concepts exhibiting high performance over a wide Mach range. Branch expertise is a blend of several areas, each contributing strongly to the advancement of scramjet engine performance and operability: ground test experimentation, computational model development and application, engine flowpath design and integration, and flight test experimentation. Our mission statement is: Conduct multidisciplinary research to advance tools and technologies for hypersonic air-breathing propulsion systems in support of national objectives.
Materials & Structures Experiments
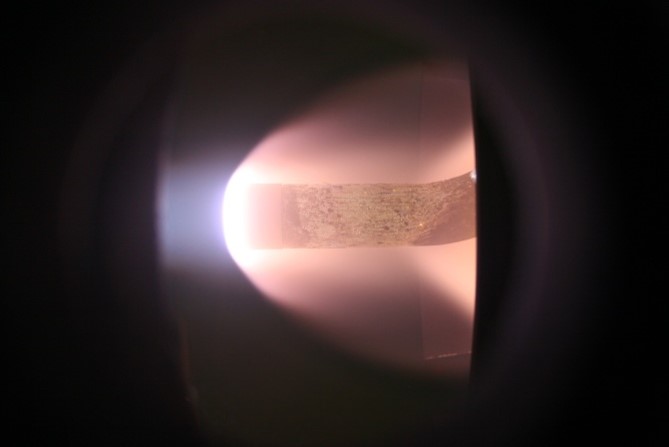
Testing the Martian Entry Vehicle in the Hypersonic Materials Environmental Test System
The Materials & Structures Experiments Branch provides the hands-on technical expertise to perform mechanical and thermal testing. The branch oversees the facilities and testing equipment. Testing capabilities cover a very wide spread of hydraulic test machines from uni-axial elevated temperature to multi-axial cryogenic temperature testing as well as operating a small research Arc-jet. Loading capabilities range from 1000 lbs to 1.2 million lbs. Testing regimes generally run from coupon through substructure to full scale test articles with the thermal capability applied to testing under 500 Kips.
Nondestructive Evaluation Sciences
The Nondestructive Evaluation Sciences Branch improves aerospace technology by pioneering advances in nondestructive measurement sciences, material state awareness, and the vehicle health monitoring through fundamental scientific research, technology development, and transferring these to the scientific and aerospace communities.
The branch conducts applied and computational research focused on the development of advanced nondestructive evaluation (NDE) and integrated vehicle health monitoring (IVHM) methodologies for assuring the structural safety and reliability of aero vehicles; advanced sensor development to enable improved sensitivity to structural performance properties critical to structural integrity; prototype systems development for field testing of advanced NDE and Structural Health Monitoring (SHM) techniques and addressing critical agency needs.
Safety-Critical Avionics Systems
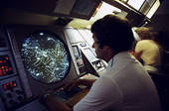
Algorithm Design and Certification for Air Traffic Separation
The Safety-Critical Avionics Systems Branch conducts research into new methodologies and tools for designing, verifying, validating, and assuring high confidence software-intensive systems to improve safety, reliability, and capacity in mission- or life-critical aero-space systems. Branch skills include formal design and verification analysis of software and hardware systems, software assurance and certification of aircraft and air traffic management systems, mishap and hazard analysis that contribute to safety improvements in current and future systems, safety analysis of avionics-based unmanned aircraft in the national airspace system (NAS), autonomy research and analysis as well as integrated experimental platform development for avionics assessment. They conduct fundamental research in developing automated formal methods, developing methods for evaluating safety-related requirements, and constructing predictive mixed human/automation models. They focus on developing and maturing safety-case frameworks, conducting research into software assurance and certification, analyzing mishaps for improved designs, developing argument-based safety assurance methods, and applying and improving integrated hazard assessment tools and methods. The Branch develops architectural principles for redundancy management and fault-tolerance, investigate avionics health management, advance mechanisms for correctly composing systems from trusted components, and improve fault modeling and emulation capabilities.
Structural Dynamics
The Structural Dynamics Branch performs basic and applied research related to the dynamic behavior of aircraft and spacecraft structures. Technical expertise includes nonlinear structural dynamics analysis and experimental methods, quantitative uncertainty-based analysis, active (“smart”) structural systems, and mechanical characterization of energy absorbing materials. Major applications areas are impact structural dynamics; including airframe crashworthiness and landing dynamics research, and space vehicle structural dynamics, with an emphasis on large and deployable space structures.
Structural Mechanics & Concepts
The Structural Mechanics and Concepts Branch conducts analyses and experiments to develop innovative structural concepts for all environments and investigate the underlying mechanics to predict the response of complex metallic and composite structures subjected to a comprehensive range of loads and temperatures. The Branch covers all speed regimes in aeronautics, from subsonic to hypersonic, space transportation systems, spacecraft and habitation concepts. They develop and demonstrate advanced light-weight structural systems and make use of new materials and fabrication processes that enable revolutionary vehicle and structural concepts. The Branch develops test techniques and capabilities that allow them to investigate structural behavior, develop understanding, and validate the theories and concepts they develop. They also conduct investigations on high temperature material systems for hot structures and atmospheric entry systems, for both Earth and Mars applications.
Structures Testing
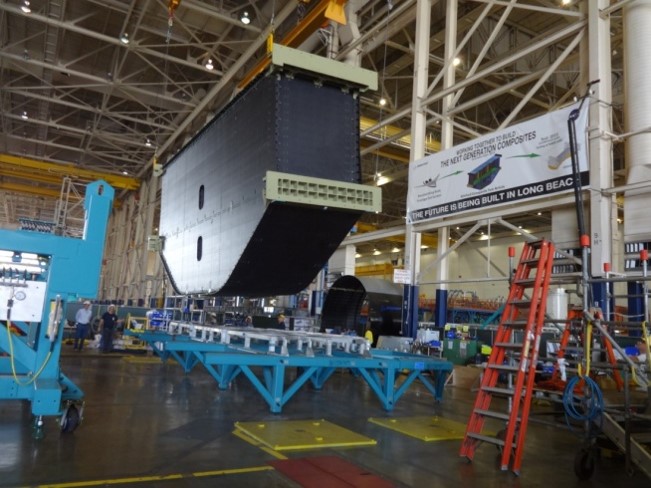
Boeing Multi-Bay Box in COLTS
The Structures Testing Branch conducts destructive and non-destructive structural testing. The Combined Loads Test System (COLTS) is a unique structural testing complex in which large curved panels and cylindrical shell structures are tested. Aircraft fuselage section components of commercial transports and space structures are subjected to combined loading conditions that simulate realistic flight load conditions. The Landing and Impact Research Facility (LandIR), a 240-foot high, 400-foot long, 265-foot wide, A-frame steel structure is used to provide crucial research test data on the crashworthiness of General Aviation (GA), commercial aircraft, rotorcraft and space craft. The flexible LandIR complex can be configured to perform various types of tests including retro-rocket landing systems and full-scale and sub-scale water landing tests.
Subsonic/ Transonic Testing
The Subsonic/Transonic Testing Branch provides comprehensive Wind Tunnel testing services for advanced aerodynamic concept development and assessment, advanced computational fluid dynamics tool validation & verification, and risk reduction for vehicle development of aircraft, rotorcraft, missile, and spacecraft systems for NASA, other US government agencies, commercial and international customers.
Supersonic/ Hypersonic Testing
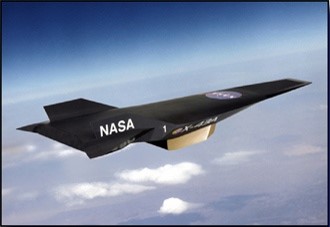
X-43 Experimental Hypersonic Aircraft
The Supersonic/Hypersonic Testing Branch operates a suite of supersonic, hypersonic and acoustic facilities. Types of research conducted are aerodynamic, aero-heating, aero-acoustics, hypersonic propulsion on flight-weight engines at true hypersonic real gas enthalpy, and real gas phenomena associated with hypersonic flight. Mach numbers range from 0.1 to simulated real gas effects at Mach 13-18, using a myriad of state-of-the-art measurement and testing techniques.
Revolutionary Aviation Technologies
The mission of the Revolutionary Aviation Technologies Branch is to identify, research, and develop innovative technologies and methodologies that will revolutionize the performance and capabilities of future air vehicles. To accomplish this, the branch: 1) investigates and matures high-risk, high-payoff technologies by conducting experimental and computational research; 2) develops, performs, assesses, and applies advanced experimental and computational methods; and 3) conducts multidisciplinary systems analysis studies to identify critical enabling technologies and to determine the system-level benefits of these technologies.